Less loss, less cooling, smaller devices
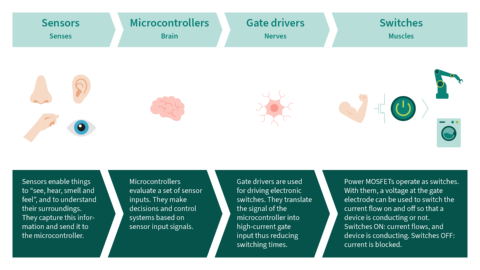
Modern power semiconductors are based on special wafer materials (silicon carbide or gallium nitride) and have specific properties optimizing energy efficiency.
For the refrigerator to cool at home, for the assembly lines to run in a factory, for the photovoltaic system to produce solar power, or for digital data to be reliably processed in a data center: Different types of collaborating semiconductors are necessary to process electricity in electronic applications in all areas of life. Sensors, microcontrollers, gate drivers and power devices are the key ingredients in applications running on electricity. They make sure that energy is converted in an efficient, reliable, smart and secured way.
New technologies have been available for several years in the field of power devices, referred to as wide-bandgap semiconductors: Silicon carbide and gallium nitride. They have many advantages in terms of energy efficiency and thus make an important contribution to decarbonization. Their structure and properties let them handle higher voltages and frequencies and still function smoothly at higher temperatures.
Infineon pioneers world’s first 300mm power gallium nitride (GaN) technology
Infineon has achieved a major milestone by developing the world's first 300mm power gallium nitride (GaN) wafer technology. As the first company to master this groundbreaking technology in a high-volume manufacturing environment, Infineon is poised to significantly drive the market for GaN-based power semiconductors.
The shift to 300mm wafers offers several advantages over the traditional 200mm wafers, including a 2.3-fold increase in chip production per wafer. Moreover, the 300mm GaN technology can leverage existing 300mm silicon manufacturing equipment, as the production processes for gallium nitride and silicon are very similar. This allows Infineon to pilot reliable GaN technology on its existing high-volume silicon 300mm production lines, enabling accelerated implementation and efficient use of capital. Once fully scaled, 300mm GaN production is expected to achieve cost parity with silicon on the RDS(on) level, making GaN products comparable in cost to their silicon counterparts. 300mm GaN is another milestone in Infineon’s strategic innovation leadership and supports the mission of decarbonization and digitalization.