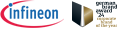
Today, hydrogen is considered the most important raw material and energy carrier for a climate-neutral industry, and the global reduction of greenhouse gas emissions. However, just as great as the potential of this versatile energy carrier are the challenges, for example in production and infrastructure. The hydrogen economy is heavily debated by both, proponents and critics. Briefly learn here everything about hydrogen and Infineon’s contribution to successful and sustainable production as well as consumption.
Hydrogen is the smallest and most common chemical element in the universe. It was discovered by the English chemist and physicist Henry Cavendish in 1766. In the periodic table, hydrogen ranks first and bears the symbol H with the atomic number 1. In its natural occurrence on earth, it is mainly found as molecular hydrogen with the symbol H2 in compounds with other elements and is found in all living organisms as well as in the raw material of all life: water (H2O).
In its pure form, hydrogen is an invisible, volatile, and odorless gas that is much lighter than air. It is a non-toxic yet not harmless gas that is highly flammable and can lead to fires and explosions in the event of uncontrolled reactions. But it is precisely this "reactivity" that makes it so valuable as an energy carrier. Another advantage: due to its abundant supply, it can be produced flexibly almost anywhere in the world.
The global reduction of CO2 emissions and a successful energy transition are two of today's biggest challenges to stop climate change on our planet. We at Infineon are convinced that hydrogen will play an important role in the energy system of the near future. Its particularly great potential for CO2 reduction is to be found in the decarbonization of the energy industry and the automotive industry, i.e., the shift from CO2-emitting energy sources and thus phasing-out energy production with fossil raw materials. The hydrogen industry plays a key role in the optimization and further development of hydrogen technologies. Three reasons why hydrogen is so important for the energy transition and is considered the energy of the future:
As a rule, hydrogen is always associated with other elements. It is found as an element in water, natural gas, or crude oil, among others. To produce pure, unbound hydrogen as an energy carrier, it must be split off using energy and special processes. Power semiconductors from Infineon already support the climate-neutral production of hydrogen through electrolysis by enabling the energy-efficient provision of electricity. The following processes are used to produce hydrogen:
The greenhouse gas emissions that are produced or not produced in the process are of particular importance. Depending on the environmental friendliness and production method of hydrogen, the following color codes are used: green, blue, turquoise, and gray.
Green hydrogen is produced, for example, in electrolysis processes that use renewable energies such as wind and solar power to produce hydrogen in a CO2-neutral manner. Since no greenhouse gases are produced in this process, it is referred to as green hydrogen. Other ways of producing green hydrogen include the gasification and fermentation of biomass and the reformation of biogas.
During chip production at Infineon, the climate-neutral production of green hydrogen as a carrier and process gas is a sustainable goal. Work on this has been underway since 2021, with the construction of the first electrolysis plant for on-site production at the Villach site in Austria. This is an important step towards advancing resource-saving production in the semiconductor industry.
Our use case on semiconductor solutions for green hydrogen shows how Infineon's innovations and technological breakthroughs help to reach climate protection targets and live more sustainably as societies.
Blue hydrogen is produced in the reforming process when the CO2 generated during production is stored or reused, and thus does not enter the environment. Storage is known as CCS (Carbon Capture and Storage): The CO2 produced is captured and stored in geological repositories, for example. By storing it, blue hydrogen is considered climate-neutral in its production.
Turquoise hydrogen is produced during methane pyrolysis. Instead of CO2, solid carbon is formed as a byproduct, which can be reused as a raw material. Just as with the reusal of CO2 produced in the reforming process, solid carbon can contribute to the decarbonization of production in the industrial sector by compensating for the use of fossil raw materials. And if the energy for the high-temperature reactor during production is obtained from renewable sources, the process is considered climate-neutral.
Gray hydrogen is produced during the electrolysis or reforming process when raw fossil materials are used for production. CO2 generated during production is released into the atmosphere without further use. If one ton of hydrogen is produced, around ten tons of CO2 are generated. Because this production is harmful to the climate, it is referred to as gray hydrogen.
A fuel cell is a galvanic cell or electrochemical apparatus that converts the energy of a chemical reaction directly into electrical energy. The generation process requires continuously supplied fuel and an oxidant – for example, hydrogen (H2) and oxygen (O2). In this case, it is called a hydrogen fuel cell. In addition, other fuels such as methanol, ethanol, natural gas, or butane are suitable for energy generation.
By design, a fuel cell consists of two electrodes: an anode and a cathode, separated by an intervening electrolyte. To generate a current in a hydrogen fuel cell, hydrogen and oxygen are fed into the circuit from the outside without interference. At the anode, the hydrogen is split into its components. In this process, each hydrogen atom emits a negatively charged electron, which travels to the cathode via an electrical conductor. In this step, the energy stored in the hydrogen is released as electricity and heat: this is how the electric current is generated to drive an electric motor. What remains are positively charged hydrogen ions (H+), which emit from the electrolyte. At the same time, oxygen molecules are supplied at the cathode, and also split into their components. The positively charged oxygen ions each take up two electrons, so that negatively charged oxygen ions are formed and, together with the hydrogen ions, water is subsequently formed (H2O). This is released as harmless "waste gas" in the form of water vapor. This way, hydrogen can be used to generate energy in a climate-neutral process.
For private motorized transport, a large proportion of people are currently using vehicles powered by fossil fuels. Globally, carbon dioxide emissions from road transport were around 18% in 2018. As part of a greener transport sector, the development of hydrogen technology is key to sustainably reducing overall emissions, alongside battery-based electromobility. As a market leader in automotive semiconductors and a thought leader for electromobility, Infineon is already supporting the automotive industry's path to a climate-friendly and carbon-free future. Infineon also offers power semiconductor solutions for the efficient use of green and blue hydrogen in the field of hydrogen fuel cells.
In European rail transport, the "Coradia iLint" passenger train from the French company Alstom is a showcase project for successful as well as market-ready operation using fuel cell technology.
Looking at the potential in various vehicle segments, hydrogen propulsion will be a preferred alternative to fossil fuel for heavy trucks and commercial vehicles over longer distances. On the high seas, too, hydrogen propulsion can be an environmentally friendly alternative for cargo ships, for example. High-performance fuel cell vehicles enabling long ranges of up to 700 kilometers already exist today: But in the future, purely battery-electric drives will be the first choice, especially for light commercial and passenger vehicles with ranges of up to 400 kilometers, while hydrogen propulsion offers a viable alternative for heavier vehicles with longer ranges. At Infineon, we are convinced that both types of drive are necessary to achieve the global CO2 reduction targets.
A fuel cell car uses hydrogen as fuel for its electric drive – the technology is also known as hydrogen mobility. No emissions are produced during energy generation, and only water vapor is emitted as exhaust gas. The number of hydrogen cars from major automotive companies is still manageable today. As far as the range of hydrogen cars is concerned, Hyundai's "Nexo" SUV, for example, can cover more than 700 km on one tank of fuel. Consumption is around 0.9 kg of hydrogen per 100 km. The trend toward hydrogen mobility is clearly on the rise and will for example be driven forward in 2022 by "Toyota Motor Europe" with the construction of a new fuel cell plant in Europe. Other manufacturers also have active development programs, such as BMW, Honda, Mercedes-Benz or Renault.
A clear difference between the two drive technologies is the overall efficiency from the fuel source to the vehicle wheels. The entire efficiency chain for locomotion is examined, from the extraction and provision of drive energy to its conversion into kinetic energy. In internal combustion engines, 17% (gasoline) to 19% (diesel) of the energy is converted into kinetic energy – the rest into thermal energy, i.e., heat. By comparison, the overall efficiency of fuel cell drives is around 32%. In vehicles with purely electric drives, there is a clear correlation between the primary energy used to generate electricity and the overall efficiency of the vehicle. If the electricity used to charge the vehicle battery is generated mainly from hydropower, as in Norway, for example, the overall efficiency is over 60%. If, on the other hand, mainly fossil fuels are used to generate electricity, the overall efficiency of the purely electric vehicle drops to values that are better than those of internal combustion engines, but hardly higher than those of fuel cell vehicles.
For the comparison between fuel cell and purely battery electric drives, it makes sense to look at the functional chain from the charging station or hydrogen tank to the wheels. As already mentioned, both drives are electric – with the difference that the chain of action is comparatively longer in the case of fuel cell drives and additional electrical energy is generated during the drive. Meanwhile in a fuel cell, energy conversion takes place in more intermediate stages, resulting in an overall efficiency of around 48%. Most of the remaining energy is converted into thermal energy. By contrast, the efficiency of purely battery-electric drives is around 75%. You can find out more about electromobility in our article “ Electromobility Guide: All you need to know.“
However, the lower efficiency of fuel cell drives has the advantage that additional thermal energy is available for heating the passenger cell in colder seasons. And unlike purely battery-electric driving, this can be done without painful losses in electric range. The hydrogen drive also scores in terms of cold-starting behavior at low temperatures.
Beside efficiency, considerations regarding installation space and weight also play an important role in the decision for the respective drive solution. Studies by "AVL" and "Ricardo" show, for example, that the fuel cell drive for a truck with a gross vehicle weight of 44 tons and a range of 800 km is the best drive solution according to these criteria. The pure diesel drive, on the other hand, scores well for longer ranges, while the pure battery-electric drive performs worst in these studies in terms of both remaining cargo space and remaining payload.
Nevertheless, when making these comparisons, it must be borne in mind that we are still at the very beginning of a new cycle of innovation in hydrogen propulsion. For example, significantly better hydrogen tank solutions can be found through new composite materials. And in terms of overall efficiency, it makes sense to combine the advantages of fuel cells by using them as "range extenders" with the advantages of purely battery-electric drive. For short and medium distances, the necessary drive energy is supplied by the drive battery alone, and for longer distances (or when overtaking) additional energy is provided by the fuel cells.
In a hydrogen drive with fuel cells, fuel in form of hydrogen and oxygen is continuously supplied to the cell block, similar to the supply of gasoline or diesel fuels in internal combustion engines. Batteries for driving electric motors, on the other hand, differ in the way they are used: They convert chemical energy stored in the cell block to generate electricity. You can learn more about batteries in our article “ Battery Management System – handling of batteries.“
One advantage of fuel cells is that they allow for continuous operation over a long period of time without intermediate charging of electrical energy. In addition, the time required to refuel hydrogen – comparable to today's internal combustion engines – is less than that required to recharge a battery.
The development of a widespread hydrogen infrastructure for mobility is one of the greatest challenges for the widespread use and establishment of hydrogen mobility. Refueling options are still limited today: The refueling station network in Germany consists of about 100 hydrogen refueling stations or H2 refueling stations, most of which are in the vicinity of large cities. Supported by a consortium of several industrial companies, they are to be expanded to around 400 by 2025. The state of the current infrastructure makes it clear that hydrogen mobility is still in the development stage. Manufacturers such as MAN and Hyundai are already working on hydrogen-powered truck series that will benefit from this development.
In the field of heavy-duty commercial vehicles, such as trucks or busses that regularly travel long distances, hydrogen propulsion can be an important step towards decarbonization. Due to the low weight of hydrogen when compared to battery weight, the fast refueling in a few minutes and the higher range compared to purely battery-powered electric engines, hydrogen drives enable efficient driving.
For hydrogen to play a key role in the environmentally friendly energy mix and the decarbonization of the economy of the future, substantial investments must be made in supply capacity and security. This includes, among other things, a rapid expansion of infrastructure, renewable energies, and the massive development of electrolysis capacities to be able to compete with fossil fuels in terms of cost. Currently, there is a demand for CO2-neutral green hydrogen in industry and transport, which Germany cannot yet meet on its own with its production of climate-neutral electricity. Global networking and the expansion of green hydrogen production capacities will be part of the solution. In addition, improvements are needed in the transport and storage of hydrogen to minimize energy losses in the long term.
At Infineon, we stand ready to support this transformation. Learn more about the opportunities and challenges in our "Infineon Paper on Hydrogen Economy."
Last update: August 2022