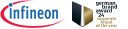
If there is a field of research considered to have revolutionary potential among scientists in the fields of computing and technology today, then quantum computing is at the top of the list worldwide – as one of the emerging key technologies of the 21st century. But how exactly is the operation mode defined, what do superpositions have to do with it, and why could a quantum computer have already analyzed all the para-graphs of this article simultaneously? We answer the most important questions and offer you a glimpse into the future.
Simply explained, a quantum computer is a processor that uses the laws of quan-tum mechanics to perform calculations. It thus enables very precise simulations of smallest physical particles (e.g., atoms and molecules), as particularly in this field quantum mechanics is significant. A principle that also helps with a multitude of questions relevant to everyday life. Its mode of operation differs from the classical computer, which is based on clearly defined electrical states: A quantum computer uses the interdependence of quantum mechanical states, which are described by means of probabilities. In order to understand quantum computing, two physical principles or states are fundamental: quantum entanglement and the superposition principle. Where classical computers and supercomputers clearly reach their limits with their state-of-the-art processors, quantum computing promises new solutions for previously unsolvable tasks – thanks to much more powerful computing capacities.
To illustrate the function of a quantum computer, let's first look at the way data is calculated in a classical computer: it follows linear calculations that take place one after the other. For example, when calculating an optimal and time-saving route sequence for visiting different locations – also known as the Travelling Salesperson Problem and a key challenge particularly in logistics and route planning. To determine the optimal route, a computer simulates all different travel routes, one after the other, taking additional factors into account, and recommends a route based on all these calculations.
How does a quantum computer proceed with this a calculation? In short: like several classical computers at the same time. This is because a quantum processor performs not just one, but several calculations in parallel and based on this, determines the optimal route more quickly. How is this possible? Quantum computers do not operate based on a classical bit (binary digit) as the smallest unit of information, but are based on a quantum bit – also called qubit. Due to the characteristics of qubits, unlike in today's computers data is not searched and processed sequentially, but simultaneously. A true miracle in terms of multitasking, which can be increased exponentially with each additional qubit.
A qubit or quantum bit is the quantum mechanical counterpart to the classical bit, and the basic unit of calculation in quantum computers. While bits are technically based on charges in electronic circuits, quantum computing currently uses different variants for quantum bits. Qubits can consist of atoms, ions, electrons or even photons, whereby different material systems can be used in each case. In some cases, additional effects such as superconductivity are used to achieve the desired characteristics.
Unlike a bit, a qubit can assume two values or states simultaneously. While a bit in the binary system assumes either the state 0 or 1, a qubit can assume both states simultaneously for a certain period. A quantum computer now has a huge advantage, because that one computing step affects all existing states simultaneously. A qubit can thus perform several calculations at the same time, and due to this special characteristic, the result is an outstanding computing performance that can be increased exponentially. In terms of qubit vs. bit, there is a clear winner in theoretical calculations – although the computing power of qubits is limited to the problem and in some cases cannot be applied. Quantum computers owe this victory to two key principles of quantum physics: quantum entanglement and superposition.
The first key principle of quantum computers is quantum entanglement: qubits are capable of connecting with each other – this is called entanglement. For example, through their magnetic fields they enter a specific kind of interdependence that makes their respective behavior conditional on each other. They are firmly connected to each other in a certain way; and in some cases, it doesn't even matter how far apart they are. So, if one qubit is changed, the qubit entangled with it takes on a matching change without any time delay and regardless of the distance – a truly astonishing regularity.
A visual example to simply explain quantum entanglement: If you influence a single qubit on the moon, all its partner qubits entangled with it on earth will also be influenced at the same time. Another simple example to explain quantum entanglement is the classic shell game – in this case with only two shells. As a neutral observer, we do not know under which shell a pea is located. In this moment, each shell has two states: "pea inside" and "no pea inside". Only when we lift one of the shells, we recognize the state and thus immediately know the result when we lift the second shell. This also works if both shells are placed in different rooms before being lifted. In the classical game, the pea has already been placed under one of the two shells before they are lifted. Surprisingly, this is not the case in quantum mechanics: the pea only decides which shell it will appear under in the moment one of them is lifted.
Quantum entanglement can also influence the computing power of a quantum computer, because it increases with the number of qubits used. The more qubits are entangled with each other, the more states can be calculated and processed simultaneously. Principally any calculations on a quantum computer can also be achieved by a sequence of different operations on only two successively entangled qubits, but this can in part require significantly more computing steps. The state of entanglement also makes computing with algorithms possible in the first place, because it makes the qubits controllable. If all qubits were completely independent of each other, computing would be impossible. It is no different to the classical computer: if you add two numbers and the result has nothing to do with which numbers you entered, the calculation is useless. It is the same with qubits: Only the dependence of the behavior enables specific calculations.
The second key principle of quantum computers is superposition: a special characteristic of qubits that allows them to assume two or more states simultaneously for a certain period (coherence time). The quantum mechanical coherence time, which can be a fraction of a second up to more than an hour, is immediately followed by the loss of superposition (and entanglement) – also called decoherence. With a single qubit this may not be impressive; it gets exciting with the addition of more qubits: With two qubits, four states are simultaneously possible, with ten already more than 1000, and with 20 even more than a million. With each qubit, the amount of information that can be stored in the system doubles. With just a few dozen qubits, quantum computers exceed the storage capacity of the most efficient classical computers. In combination with entanglement, which makes it possible to compute on all these states at the same time, it becomes quite an efficient system.
This also solves the two questions of the introductory text, namely what superpositions have to do with the operation mode and why a quantum computer would have read through this article already: due to superposition, a quantum computer could in theory have analyzed every paragraph of this article at the same time and just as quickly drawn its conclusions from it. Another example of superposition is the participation of a quantum computer in a lottery draw. Whereas we would have to fill out a huge number of lottery tickets to have a good chance of winning the main prize (statistically around 1 in 140 million), a quantum computer leaves nothing to chance and always has six correct numbers and the super number with a single ticket. Almost magically, the right crosses always appear when you pull the lottery ticket out of the envelope. A very talented lottery player, isn't it? The superposition principle thus holds enormous potential in many areas and provides an initial explanation for the worldwide race to developing the most efficient quantum computers.
For decades, the computer within your device with which you are reading this article right now was only a theoretical assumption. And the worldwide race to develop the most efficient computer is still in full swing. Today’s situation with quantum computers is similar, for which, despite initial successes, widespread use will probably still be many years away. So, can quantum computers in future become universally accepted faster than expected like once computers were, and are there already quantum computers that have made their way beyond theory? Let's look at the quantum computer and its history.
The first quantum of knowledge about how quantum mechanics can be used for calculations was first described toward the end of the 1950s and has been further developed by many scientists across the decades – we start with the doubts of a well-known German physicist, who played a major role in developing quantum theory:
In the same year, the start-up "Alpine Quantum Technologies" was founded in Innsbruck around the quantum computing pioneers Reiner Blatt and Peter Zoller, in order to bring ion trap-based quantum computers to market maturity.
In addition, the Finnish start-up "IQM" is launching a superconducting platform – now one of the best-funded European companies in this field.
In the same year, the researchers at Google set another milestone: with a chip called "Sycamore", which has 53 qubits, they now claim proof of quantum supremacy – the quantum superiority over classical computers – based on a complex calculation of random numbers. Sycamore solved this task in 3 minutes and 20 seconds – while the most efficient supercomputer at the time would have taken an estimated 10,000 years. For the time being however, as it is only a feasibility study to demonstrate the computing power which is not yet suitable for practical use.
After decades of theory, the technological state of quantum computers is now ready for practice and application-based trials. Nevertheless, the technology is still in its infancy. Private individuals cannot buy their own quantum computer yet – but there are already access options via cloud systems, which are even free of charge for simple questions. Even if a lot of this is still up in the air, the race for the next digital and industrial revolution has been gaining traction for years and Germany is also participating in this development. To this end, the German government under Angela Merkel has released 2 billion euros in 2021. With a clear goal: by 2026, German quantum computers should have at least 100 individually controllable qubits that can be scaled up to 500 qubits. A sensible foundation for the future, as the development of supercomputers is slowly but surely reaching its physical limits. In addition, Germany already is an internationally important supplier of quantum technology components, such as optical quantum sensors, lasers or laboratory equipment.
Another realistic step to realization is the combination of normal computers with quantum computers to lower the threshold for application. The Canadian company D-Wave has been working in this direction for several years. Here, a so-called quantum annealer (a restricted version of a quantum computer) performs specific calculations, while the classical computer oversees the remaining calculations. VW has already tested such mixed computers containing 1,135 qubits, for example navigation systems that can be used to optimally control traffic.
Be it for the development of medicines, new materials, sophisticated logistics or financial processes as well as artificial intelligence and machine learning: sooner or later, quantum computing and its advantages will determine the future.
With their operating power and parallel calculation methods, quantum computers can process huge amounts of data particularly quickly and efficiently. The experiment by Google and its quantum computer "Sycamore" proved its superiority (quantum supremacy) over computers in 2019: What took their 53-qubit quantum computer just 3 minutes and 20 seconds to do would have taken the most powerful supercomputer an estimated 10,000 years.
Five exemplary areas for which quantum computers and their advantages will be important are:
Especially for scientific disciplines, the performance of quantum computers represents an enormous potential that today's computers or supercomputers will not reach.
If we compare quantum computers with classical computers, they differ primarily in the way they calculate data. While a computer is based on bits as the smallest unit of computation, quantum computers are based on quantum bits, also called qubits. The characteristics of qubits enable a much more powerful calculation for specific questions, because unlike bits, they can assume different states at the same time. In addition, the computing power of a quantum computer can be increased exponentially with each additional qubit.
So, have quantum computers won the comparison with computers? If it's purely a matter of computing power and you limit yourself to applications in which many different computing paths play a role: Then yes! But when it comes to everyday usability, quantum computers are only ahead in theory. Because until there are practical quantum computers that will replace computers in their areas of application, great technological progress is required. Progress that will probably first and foremost make quantum computers competitive with supercomputers in scientific or research-related areas. In addition, classical computer architectures will remain the better choice in many areas. In the future, quantum computers should therefore be seen as a supplement to classical computers – they will not replace them.
To a large extent, the potential areas of application for future quantum computers correspond to those of today's supercomputers: the particularly complex calculation of simulations in the natural sciences. Here, a supercomputer follows calculations like a normal computer, but these are not calculated by a single computer but by a large, interconnected system. Such a system consists of hundreds of thousands of computing cores or computers. In addition to the CPU (Central Processing Unit) components intended for general computing tasks, these also use GPU (Graphical Processing Unit) components or other accelerators that reduce the power consumption per computing unit.
The calculation method of supercomputers is therefore based on an extremely large number of parallel calculations by several computers. The decisive difference between a quantum computer and a supercomputer is that it performs the calculations in parallel in only one system at a time.
In the duel of quantum computer vs supercomputer, the quantum computer has now won, hasn't it? As with the comparison with computers, only theoretically and for specific fields. Because there is still no concrete application for which a quantum computer has had a groundbreaking success that beats supercomputers. The Google task, which took a quantum computer only 3 minutes and 20 seconds and a supercomputer an estimated 10,000 years, was objectively a "home field advantage" for the quantum computer's method of calculation. The solution was more a demonstration of feasibility than an actual application. Perhaps comparable to the Sputnik satellite, which in 1957 was the first satellite in space that could do nothing other than announce by radio: "I am here". So, as long as there are no quantum computers that can be used in practice, supercomputers will continue to be of the utmost importance for science in all fields. But even for a quantum computing age, it can certainly be assumed that both computer species will coexist.
The development of quantum computers poses different challenges for research and society today: On the one hand, the technical feasibility of development and, on the other hand, the possible global impact on digital security.
1. Technical challenges of quantum computers
One of the greatest challenges for scientists lies in the basic technical conditions for making the computing power of a quantum computer usable or controllable in the first place. This is due to the extreme sensitivity of qubits: even the slightest external impact can cause them to lose their specific quantum characteristics. Vibrations, magnetic and electric fields, light, temperatures and many other external influences cause errors and can destroy the computing process. In order to keep the qubits stable, they must be well shielded, but it must also be possible to manipulate them to be able to perform a calculation at all. This is like squaring a circle. To achieve this, superconducting qubits, for example, are cooled to temperatures close to absolute zero of –273.15°C and shielded several times from the environment; but even then, this qubit species only ever remains stable for fractions of a second – a minimal time period during which calculations have to take place.
Other qubit technologies such as ions, on the other hand, are much more stable and retain their properties over minutes or even hours, but they take much longer for a single computing step. The main focus of current development is:
And of course, there is no established software for something that does not yet exist. Quantum computers function with completely different laws than today's computers, which leads to completely new challenges for the software. At this point quantum algorithms are a field that is often overlooked. The fantastic ability of a quantum computer to calculate simultaneously on an unbelievably large number of states is not so easy to use beyond theory. The goal of a calculation is the correct result. But if all the states of the quantum computer had to be read out for this, not much would be gained, because it is a huge number. Therefore, specific algorithms are needed to ensure that only the correct state with the greatest probability remains at the end of a calculation. This is a task that has so far only been solved for a manageable number of problems.
These challenges make it clear how far quantum computers are from being used in our everyday lives.
2. The challenge of digital security through quantum computers
In addition to the technically demanding basic requirements for the development of quantum computers and their application, society will also have to adapt to the capabilities of this technology as soon as quantum computers can be used on a larger scale. For it is precisely in the area of encryption, and thus digital security, that special challenges can arise in both the private and professional spheres.
"The digital lockpick of the future" – that could be a headline when it comes to quantum computers and their ability to crack widespread encryption systems in use today. The crypto procedures that make surfing the net secure today must be strengthened in the future and be able to withstand the computations of quantum computers. And also, because supercomputers are becoming more and more powerful, and today's encryption methods are only secure because it is simply computationally too intense to decrypt them over several years.
Quantum computers can crack older systems in the future. However, only if the older systems remain old and are not replaced by new encryption methods. This is what post-quantum cryptography is working on, researching quantum-safe encryption methods. As a pioneer, Infineon is actively pushing this research– you can read more about it in our article on post-quantum cryptography.
Edward Snowden's revelations in 2014 showed just how great the interest can be: the National Security Agency (NSA) was already conducting intense research on the use of quantum computers in cryptography. Post-quantum cryptography is already displaying initial solutions and successes for these challenges. As with conventional computers, it is up to us humans to decide what potential quantum computers will develop in the future and what they will be used for.
Will we soon be able to buy a quantum computer? Will smartphones become much smarter? The status quo of quantum computers promises groundbreaking developments, which will however be reserved primarily for scientifically complex areas. This is mainly due to the technical effort required for the operation and error-free functioning of a quantum computer. And because of the specific areas of application in which, according to the current state of knowledge, a quantum computer can only fully exploit its great potential. All this makes quantum computers still a vague vision of the future for private users.
From the financial sector, logistics and transport processes to material development and complex simulations, quantum computers have the potential for revolutionary developments. A global race is underway to successfully commercialize this technology, and since the very beginning Infineon has played a supporting role by contributing to its development.
A significant focus is on the scalability of quantum computing technology, which will help shape our digital future. With know-how and competencies in relevant areas from chip design to materials, production and software, Infineon will advance the development of quantum computing. To take quantum computing from development and theoretical foundations to commercially relevant applications, Infineon is also a founding member of QUTAC – the consortium of leading German companies to bring quantum computing to the level of large-scale industrial application, and to promote the emergence of a commercially successful and sovereign quantum computing ecosystem.
For a detailed insight into Infineon's technological support, read this article: Quantum computing – game changer for tomorrow.
Last update: November 2021